The advanced artificial systems represented by inorganic semiconductor electronic devices have carried the human information age.
However, their planar two-dimensional geometric configuration and rigid mechanical properties lead to an inability to perfectly integrate with three-dimensional and soft biological tissues.
This huge gap in geometry and mechanics poses a great obstacle to the development of bio-integrated microsystems.
However, behind this global problem, there is a broad application prospect that can revolutionize the field of human medical care.
Luan Haiwen, an assistant professor at the University of California, San Diego, is dedicated to the research of bio-integrated microsystems.He is dedicated to the research and development of a new generation of multifunctional devices for bio-integration, exploring how to closely interact complex artificial systems with biological organisms, in order to bring more reliable and convenient digital biomedical technology to the general public, thereby promoting medical innovation and life science research.
Advertisement
Around this direction, he has developed a set of structural design, analysis simulation, and preparation methods guided by solid mechanics theory, for the design and preparation of high-performance bio-integrated devices.
This mainly includes three levels: wearable flexible electronic devices at the individual level; three-dimensional intelligent multifunctional bio-integrated interfaces at the cellular and tissue levels; and reprogrammable mechanical metamaterial systems at the material and structural levels.
By integrating intelligent electronics and microfluidics technology into life systems, he has created multifunctional bio-integrated micro-scale systems for addressing medical challenges, promoting biomedical research from multiple dimensions such as drug screening, therapy exploration, and artificial organ cultivation. Luan Haiwen has become one of the Chinese inductees of the "35 Innovators Under 35" in the 2023 MIT Technology Review.Guided by mechanical analysis, the development of three-dimensional micro-scale structure assembly technology is emphasized.
As mentioned above, previously, it was very challenging to perfectly integrate two-dimensional electronic devices with three-dimensional soft biological tissues for the purpose of electrophysiological monitoring of the latter.
Three-dimensional microstructures with open networks are ubiquitous in the biological world. Therefore, Luan Haiwen and his collaborators hope to better integrate with biological tissues by designing and preparing some three-dimensional micro-scale electronic devices.
"We once thought of using 3D printing technology, but it is currently difficult to fabricate high-performance electronic devices with it," Luan Haiwen stated.After extensive investigation and research, they proposed a concept: could high-performance two-dimensional electronic devices be reconstructed into three-dimensional structures by thinning the material and applying compression?
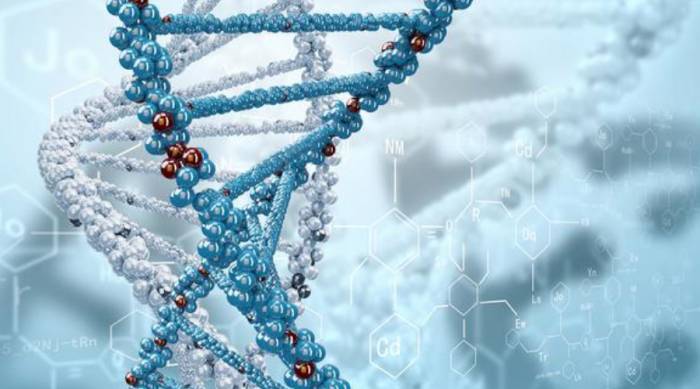
In their view, although the silicon material used for preparing semiconductor electronic devices is inherently hard and brittle, if it is thin enough, it will become very soft and easy to deform, and it is less likely to break during bending.
However, brittle materials, even if very thin, still cannot withstand tension or torsion. One of the previous ideas for designing inorganic stretchable electronics was to pre-compress the thin-film material into a wavy shape, endowing brittle materials with stretchable mechanical properties through the geometric shape of compression bending. This method of compression bending can induce the transformation of two-dimensional structures into three-dimensional shapes, inspiring the design and preparation of three-dimensional microstructures.
"That is to say, by introducing the corresponding compressive stress locally in the two-dimensional structure, it can be induced to produce compression bending, which allows for controlled transformation from a two-dimensional structure to a three-dimensional structure, and thus the preparation of three-dimensional flexible high-performance electronic devices," explained Luan Haiwen.
And this is also the core of the three-dimensional microstructure assembly technology [1-3].Luan Haiwen points out: "This technology has a strong versatility, not only can be used for various inorganic materials and organic materials, the material itself can be soft or hard, and is also applicable to nano, micrometer, centimeter, decimeter, and various other scales."
It is worth noting that mechanical analysis and structural design play a key role in this process.
Firstly, materials that are inherently very hard can be transformed into soft and stretchable device systems.
Secondly, it can guide the controllable compression buckling deformation from two-dimensional to three-dimensional. Mechanical analysis can accurately predict the final three-dimensional shape.
Thirdly, it can guide the reconfigurable metamaterials to achieve precise switching of different shapes.However, in the development of this technology, the research team also encountered many challenges.
Firstly, how to design various high-performance three-dimensional electronic devices.
This is because, the structural transformation from two-dimensional to three-dimensional is restricted by the two-dimensional structure, which makes it impossible to form certain three-dimensional structures. Therefore, constructing as many geometric structures as possible based on this technology is one of the main tasks of Luan Haiwen during his doctoral studies.
Secondly, while applying this technology to electronic devices, whether it can also be extended to other fields, such as the design and preparation of three-dimensional microfluidic networks.
Thirdly, how to find a relatively unique biological integration application scenario for the three-dimensional electronic devices prepared based on this technology.In this regard, Luan Haiwen and his collaborators have developed a three-dimensional flexible multifunctional neural interface capable of gently wrapping around cerebral cortical organoids [4,5].
They primarily used mechanical design methods to create complex three-dimensional structures that match the shape of cerebral cortical organoids, and then used them to drive electronic devices including sensing and stimulation functions, gently adhering to the surface of the cerebral cortical organoids to achieve comprehensive electrophysiological monitoring of the latter. It is worth noting that the spatial distribution of devices such as microelectrodes can be accurately predicted by mechanical analysis.
It is understood that this neural interface has, for the first time, enabled the observation of neural activity across the entire surface of a cerebral organoid, providing a required platform for studying, understanding, and treating neurological diseases and injuries, thereby bringing better solutions for brain repair.
At the same time, the three-dimensional neural interface can also be used to combine two different cerebral organoids to study the functions and connections between different brain regions.
In fact, in three-dimensional biological interfaces, in addition to electronic components used for sensing and regulating biological behavior, there is also a need for a "lifeline" that can support larger and more complex biological tissues, to transport nutrients and eliminate waste.Therefore, based on the guidance of mechanical analysis and structural design, a complex three-dimensional microfluidic network assembly method has also been developed [6].
Luan Haiwen pointed out that this method is an important feasible approach to creating an intelligent three-dimensional microvascular system that integrates transportation with sensing/actuation. Compared to previous technologies such as 3D printing and laser ablation, it has at least the following advantages:
Firstly, it can simultaneously achieve local high-precision dimensions (such as a channel width of 2 micrometers) and overall large dimensions.
Secondly, it can mimic the size and function of capillaries.
Thirdly, it can integrate high-performance electronic devices for complex sensing and control.With these three advantages, this method has a relatively broad application space. In addition to being able to achieve important applications in the fields of bioanalysis and drug delivery, it is also suitable for preparing complex artificial vascular networks. Luan Haiwen said.
Dedicated to the development of bio-integrated devices, committed to promoting medical innovation and life science research
Luan Haiwen was born in Yantai, Shandong, and his parents are university teachers in electrical and electronic engineering and mechanical engineering, respectively.
The parents' professions have had a subtle influence on Luan Haiwen's growth, helping him to cultivate some good study habits from a young age. For example, having a strong curiosity about new things, liking to ask questions actively, and recording new ideas and knowledge in notes in a timely manner.During his middle school years, Luan Haiwen developed a keen interest in architecture, and the Wenchuan earthquake in 2008 made him realize the importance of civil engineering. Consequently, after the college entrance examination in 2009, he chose to apply for the civil engineering program at Tongji University and was successfully admitted.
After graduating with a bachelor's degree in 2013, he went to Northwestern University in the United States, where he spent three significant periods of research: master's, Ph.D., and postdoctoral studies.
During his master's program, he continued to study civil engineering, under the guidance of Professor Huang Yonggang, a member of three academies in the United States. He focused on deepening his knowledge in the direction of mechanical analysis and structural design, hoping to build strong and reliable homes for the people in the future.
Under Professor Huang's guidance, he gradually found that mechanical analysis and structural design can play an irreplaceable role in the field of micro-nano electronic device processing, especially in the processing of stretchable electronic devices. These two skills happened to be his expertise during his undergraduate studies. Therefore, he focused on the research of mechanical properties of micro-scale stretchable electronic devices.
During his Ph.D. program, he pursued solid mechanics in the field of mechanical engineering, studying the guidance of mechanics in the assembly of three-dimensional micro-scale structures and their applications in the field of bio-integrated devices. He would use finite element simulation and analytical mechanical models to explore related topics.Because these studies are closely related to structural design, his previous experience in studying civil engineering has also been of great help to him.
"Through mechanical research, the series of three-dimensional electronic biological interfaces I have developed can bridge the huge differences in physical properties, allowing for a closer interaction between electronics and biology, thereby advancing research in the field of biomedicine," said Luan Haiwen.
During his postdoctoral stage, Luan Haiwen studied experimental skills related to bio-integrated micro-nano devices under the guidance of Professor John A. Rogers, a member of the American Academy of Sciences.
He not only mastered knowledge in various aspects such as preparation, characterization, and maintenance but also excelled in applying the theoretical and computational skills he learned during his graduate studies. This has made him a researcher capable of independently conducting a full range of research in the field of bio-integrated micro-scale devices.
Additionally, it is worth mentioning that he has also worked part-time and provided technical consulting services in a startup company co-founded by Academician Rogers, and participated in the development of skin-integrated flexible and stretchable wearable devices.It is understood that such devices, relying on common smartphones/tablet computers, can provide intensive care level health signal monitoring, such as uninterrupted health monitoring for premature infants.
In March 2024, he joined the University of California, San Diego as an assistant professor.
At present, he plans to integrate the theoretical, computational, and experimental skills he has learned to carry out his own independent research projects.
For example, developing new mechanics theories to guide the assembly of three-dimensional microscale structures and other basic research, to promote the development of the next generation of three-dimensional microelectronics technology and three-dimensional microvascular networks, and to better contribute to life sciences and the medical field.
Comments